How do cetaceans and other marine vertebrates avoid decompression sickness? A new explanation for beating the bends.
By Nick Martinez, SRC intern
The challenge of decompression sickness (DCS) and Nitrogen Narcosis, have always proved a threat to the deep diving vertebrates of the marine world. For years, scientists have debated over how cetaceans and other marine vertebrates are able to avoid DCS. In a recent paper from Daniel Parraga and colleagues, Pulmonary ventilation – perfusion mismatch: a novel hypothesis for how diving vertebrates may avoid the bends, the authors introduce a new hypothesis attempting to explain how this feat is actually performed.
To counter the challenges of DCS and nitrogen narcosis, cetaceans have developed a variety of adaptations that allow them to avoid catastrophic effects while diving. Many of the large deep diving cetaceans share a smaller mass-specific total lung capacity than that of shallower divers (Parraga et al. 2018). What this means is that larger whales have a comparatively smaller lung to body size ratio than that of dolphins, for example. Having a smaller mass-specific total lung capacity allows these cetaceans to have a smaller portion of their thoracic volume to be taken up and are able to have a larger vascular network. This vascular network comprises of multiple layers of alveoli, allowing there to be more oxygen storage when taking air at the surface. Smaller lungs prove more advantageous because a larger lung size would allow for more N2 absorption, increasing the risk of DCS (2018). Cetaceans also have a network of cartilaginous reinforcements that maintain the patency of airways during dives (2018). This system of cartilage allows for cetaceans to have high respiratory flow when taking breaths at the surface. The most important feature of the cartilage involves the facilitation of alveolar collapse at specific depths. Facilitating alveolar collapse prevents any excess N2 absorption into the bloodstream and thus reduces the risks of DCS (Figure 1). Finally, cetaceans have a series of vascular plexus that aid in oxygen storage and the redirection of air volume to the sound producing areas of the organism. This system of vascular control is the framework for complete DCS and nitrogen narcosis prevention. However, to understand exactly how cetaceans use their adapted bodies to prevent DCS and nitrogen narcosis, it is important to understand how they regulate gas exchange at depth various depths.
In Parraga’s paper, the author introduces the importance of gas exchange in marine vertebrates by first introducing two variables: VA and Q. VA equates to the rate of alveolar ventilation (air reaching the alveoli) while Q tells how much blood is perfusing the alveoli. These two variables are important for understanding how cetaceans are able to manage the rate of gas exchange within their body. If, for example, there were no management of gas exchange while diving, any air breathing organism would immediately fall to the effects of DCS because of the amount of nitrogen gas that is being dissolved into the blood stream. Originally, scientists believed that cetaceans controlled gas exchange by performing passive compression of alveoli and airways at specific depths. However, Parraga et al. note that this passive compression at significant depths does not explain how cetaceans prevent nitrogen related catastrophes at shallower depths. For example, many dolphins and small whales who get caught in gill nets have been found dead with signs of unregulated gas exchange, proving that regulated gas exchange is crucial at any depth. In fact, Parraga notes that between different species, alveolar collapse depth is completely variable between species.
The authors propose a new hypothesis attempting to explain how cetaceans are able to avoid DCS and nitrogen intake at any depth. This new hypothesis describes how cetaceans are able perform alterations in alveolar ventilation (VA) and perfusion (Q) that allows selective gas exchange during natural (dives not at alveolar collapse depth) and deep dives (at collapse depth). The way cetaceans perform this unique adaptation is they alter the VA/Q ratio to reduce inert gas uptake while simultaneously exchanging some O2 and CO2 (Figure 2) (Farhi et al. 1967). Altering the gas exchange ratio requires these organisms to force pulmonary gas into higher and lower parts of the lung. In doing so, a manual shut off of specific air ways occurs and it prevents blood from accessing air that would otherwise contaminate it. While unique, this process is very susceptible to failure provided that the right environmental and/or behavioral stressors are introduced to the organism.
Like all research, this information allows scientists to analyze exactly how these fragile organisms fall susceptible to anthropogenic factors. For example, beaked whales near areas of Naval sonar testing have been found stranded on beaches with gas bubbles in their blood stream (Jepson PD et al. 2003), proving that even in shallow waters, the sonars were able to disrupt the whales’ ability to manage gas exchange. The research conducted by Parraga and colleagues, and by other scientists, therefore proves vital in protecting these delicate species around the world. By understanding how cetaceans operate at various depths, scientists can turn research into policy that would help protect these organisms from population decline.
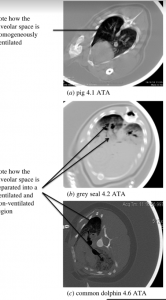
Figure 1: This figure displays a cross sectioned X-ray image of a pig (a), a grey seal (b) and a common dolphin (c) in a pressurized hyperbolic chamber. It is clear to see that the gas distribution of each organism at the same depths is different. In the pig, gas distribution stays uniform throughout but in the dolphin and seal, the air is transferred to the upper parts of the lung.
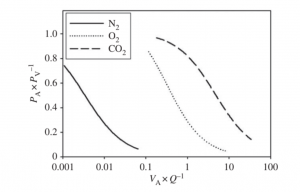
Figure 2: this figure displays the variations in the gas exchange ratios for each gas. The equation shows how much gas exchange is going on with 0. As gas exchange ratios increase, the amount of gas that is actually being exchanged, decreases.
References
Garcia Parraga, Daniel, Moore, Michael J., Fahlman, Andreas, Pulmonary ventilation perfusion-mismatch: a novel hypothesis for how diving vertebrates may avoid the bends, proceedings of the Royal Society B: Biological Sciences 285 (2018)
Farhi LE, Yokoyama T. 1967 Effects of ventilation-perfusion inequality on elimination of inert gases. Resp. Physiol. 3, 12 – 20. (doi:10.1016/0034-5687(67)90019-9)
Jepson PD et al. 2003 Gas-bubble lesions in stranded cetaceans. Nature 425, 575 – 576. (doi:10.1038/nature 02528, 2004)