Loss of North American Freshwater Biodiversity
By Chris Schenker, SRC intern
Despite garnering less attention than their marine counterparts, freshwater species are diverse, important, and also under threat. Despite covering only 0.8% of the world’s surface and accounting for about 0.01% of the world’s water, freshwater contains at least 100,000 distinct species (Dudgeon et al. 2006). Comparisons to other environments are difficult because of the variation surrounding estimates of the total number of species on Earth. However, given freshwater’s disproportionately small share of total volume and surface area, it is beyond doubt to assert that its species density is staggeringly high. Although the interrelationships are not completely understood, freshwater biodiversity plays a crucial in ecosystem function as a whole. Despite the value that freshwater flora and fauna add from a scientific, commercial, aesthetic, cultural, and recreational perspective, the species extinction rate is far higher than that for their terrestrial counterparts (Dudgeon et al. 2006).
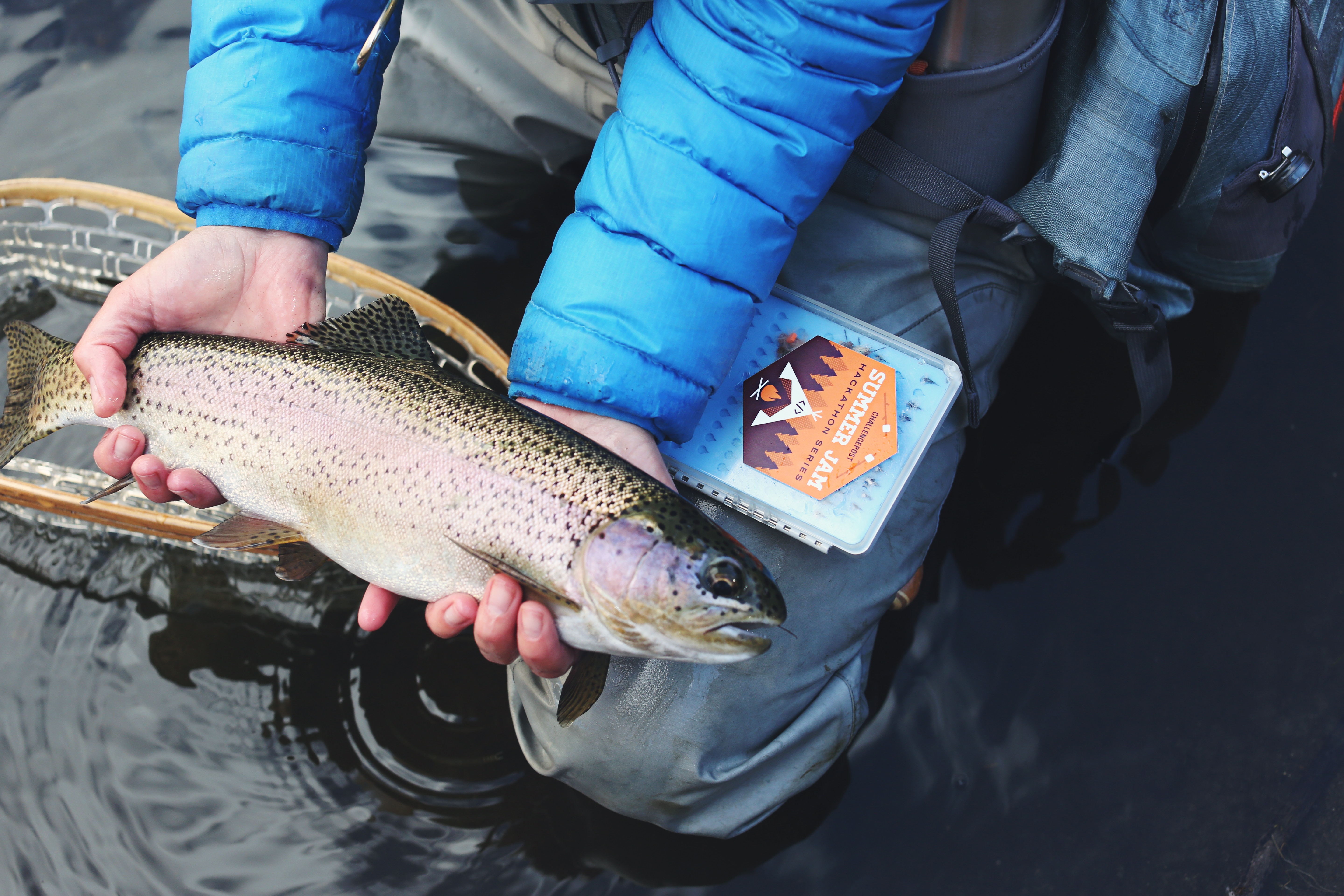
Figure 1: Freshwater fish are under threat worldwide, but the trend is especially pronounced in North America. (Unsplash.com)
This dynamic is especially clear in North America, where aquatic environments are well-studied. Although freshwater biodiversity is higher in the tropics, North America has the highest nontropical species diversity (Lundberg et al. 2000), totaling 1,213 as of 2010 and accounting for 8.9% of the world’s freshwater fish species (Nelson et al. 2004). There is a long history of species documentation in North America, with the first observed extinctions occurring in the early twentieth century. Since then, 39 species of North American freshwater fish have been declared extinct (IUCN 2011), yielding a continental extinction rate of 3.2% (Burkhead 2012). This is a higher total than is observed globally, but that is only because of North America’s longer history of continuous faunal study in comparison to the tropics. Regions with similar histories of scientific documentation display similar trends, with 3.4% of species considered extinct in Europe (Freyhof and Brooks 2011), and 3.2% of species considered extinct in the European basin (Smith KG and Darwall 2006).
The metric that really matters is the modern extinction rate in comparison to the background extinction rate. The background extinction rate, often abbreviated BER, is the taxonomic extinction rate over geologic timescales before the introduction of human pressures. Knowing both values allows scientists to calculate the ratio of modern extinction rate to background extinction rate (M:BER). There are difficulties in calculating both rates, so numbers should be interpreted with a grain of salt, but this ratio gives a rough estimate of how much faster extinction is occurring due to humanity’s global remodeling. The M:BER ratio for North American freshwater fishes has been calculated to be as high as 877 (Burkhead 2012), making it the highest number for any contemporary vertebrate group (Barnosky et al. 2011). Despite the large number of estimates and assumptions underlying this number, it highlights the severity of the problem species are facing. Fishes labeled as threatened, endangered, and declining will be subjected to the most intense pressure, especially those that are endemic and lie in the path of future human expansion.
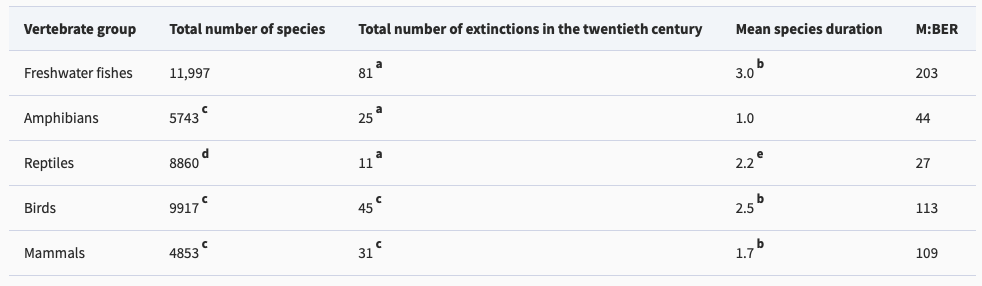
Figure 2: This table compares the modern extinction rates for world vertebrates to their estimated background extinction rates. (Burkhead 2012)
As imperiled as freshwater fishes are, they are only the beginning when it comes to declining freshwater flora and fauna. Many molluscs, such as snails and mussels, are going extinct at a rate that is an order of magnitude greater than that of freshwater fishes (Burkhead 2012). The main cause of this plight is human alteration of rivers and lakes, along with runoff, pollution, and the introduction of invasive species, such as zebra mussels (Cope et al. 2008). Mussels play an important role in their aquatic communities, such as providing habitat, filtering the water column, and depositing nutrients into the sediment. There is much scientific interest in what will happen to freshwater ecosystems as mussel species diversity and biomass declines.
The effect that mussels have on water filtration and waste recycling can vary widely based on the species, abundance, and environmental conditions present at the time. When water flow is low, such as in late summer, mussel communities can filter the entire volume. When water flow is high during times of melting and drainage, however, their effect is greatly reduced (Vaughn et al. 2004). Filtration performance is further affected by water temperature, with each species having its own optimal temperature. This means that in aquatic environments with multiple species of mussels, the ecosystem wide impacts of their filtration will vary widely based upon the relative dominance of each species, water flow, and temperature. Further complicating the equation is the fact that mussels also excrete ammonia, and their excretion rates can move in tandem with filtration rates, in the opposite direction, or not at all. It is all temperature dependent (C.C. Vaughn 2010). The ammonia excreted by mussels plays an important role in lake and riverine ecosystems, boosting primary production and also benefiting consumers down the line (Spooner and Vaughn 2006). Even if excretion and filtration rates can be modeled for a set of known variables, it is important to remember that mussel communities can change in biomass and species dominance over time, and hydrological conditions are also dynamic.
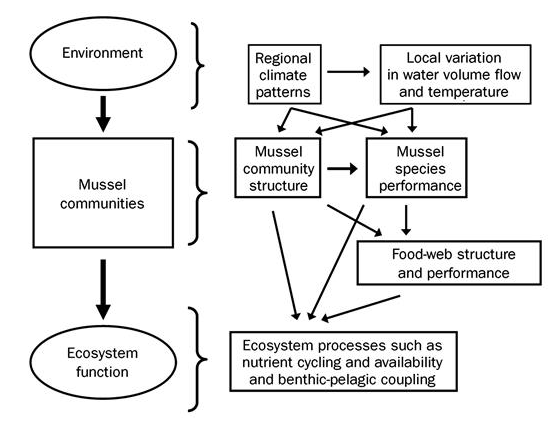
Figure 3: This conceptual model demonstrates the complicated interplay between mussel species density, community structure, and ecosystem processes. (C.C. Vaughn 2010)
This example serves an important point. Attempting to understand how an aquatic environment will respond to changes in species composition is difficult. With so much uncertainty about the effects of biodiversity loss in North American freshwater ecosystems, we as a society have no idea what we may be getting ourselves in for. It is therefore prudent to prioritize the conservation of at-risk populations so that we do not have to contend with the damage that their losses may entail.
Works Cited
Dudgeon, David & Arthington, Angela & Gessner, Mark & Kawabata, Zen-Ichiro & Knowler, D & Lévêque, Christian & J Naiman, Robert & Prieur-Richard, Anne-Hélène & Soto, D & Stiassny, Melanie & A Sullivan, Caroline. (2006). Freshwater Biodiversity: Importance, Threats, Status and Conservation Challenges. Biological reviews of the Cambridge Philosophical Society. 81. 163-82. 10.1017/S1464793105006950.
Lundberg JG Kottelat M Smith GR Stiassny MLJ Gill AC. 2000. So many fishes, so little time: An overview of recent ichthyological discovery in continental waters. Annals of the Missouri Botanical Garden 87: 26–62.
Nelson JS Crossman EJ Espinosa-Pérez H Findley LT Gilbert CR Lea RN Williams JD. 2004. Common and Scientific Names of Fishes from the United States, Canada, and Mexico, 6th ed.
American Fisheries Society. Special Publication no. 29.
[IUCN] International Union for Conservation of Nature. 2011. IUCN Red List of Threatened Species, version 2011.2. IUCN. (13 June 2012; www.iucnredlist.org)
Freyhof J Brooks E. 2011. European Red List of Freshwater Fishes. Publications Office of the European Union.
Smith KG Darwall WRT eds. 2006. The Status and Distribution of Freshwater Fish Endemic to the Mediterranean Basin. International Union for the Conservation of Nature. (14 June 2012; http://data.iucn.org/dbtw-wpd/html/Red-medfish/cover.html)
Noel M. Burkhead, Extinction Rates in North American Freshwater Fishes, 1900–2010, BioScience, Volume 62, Issue 9, September 2012, Pages 798–808, https://doi.org/10.1525/bio.2012.62.9.5
Barnosky AD et al. .2011. Has the Earth’s sixth mass extinction already arrived? Nature 471: 51–57.
Cope WG et al. 2008. Differential exposure, duration, and sensitivity of unionoidean bivalve life stages to environmental contaminants. Journal of the North American Benthological Society 27: 451–462.
C.C. Vaughn. Biodiversity losses and ecosystem function in freshwaters: emerging conclusions and research directions. Bioscience, 60 (2010), pp. 25-35, 10.1525/bio.2010.60.1.7
Vaughn CC Gido KB Spooner DE. 2004. Ecosystem processes performed by unionid mussels in stream mesocosms: Species roles and effects of abundance. Hydrobiologia 527: 35–47.
Vaughn CC Spooner DE. 2006. Unionid mussels influence macroinvertebrate assemblage structure in streams. Journal of the North American Benthological Society 25: 691–70