By Laurel Zaima, RJD Intern
ABSTRACT
Commercial shrimp fisheries’ main objective is to maximize their target catch in order to make the most profit. Commercial shrimp fisheries satisfy this goal by the utilization of trawl nets. Trawling is a very destructive form of fishing, and unfortunately, does not take into consideration ecological consequences. Trawling, by the use of beam or otter trawls, is used to maximize their catch of shrimp; however, it also maximizes their landings of by-catch. The amount of by-catch far out weighs the amount of shrimp and target species caught in trawls. The majority of by-catch, which comprise of non-commercial fish, juveniles, and benthic debris, are landed and discarded back into the ocean dead. The dumping of by-catch then contaminates the water and contributes to the death of other species living in the area. As the weighted trawl nets are dragged across the ocean floor, the benthic habitat is destroyed and collected by the net. Trawling destroys complex habitat structures and seagrass beds, and changes the biochemical composition of the sediment. The alterations to the substrate and damages to habitat configuration can induce an ecological shift of the organisms that live in that area. Invertebrates are especially sensitive to the change of the benthic habitat, and therefore, are indirectly affected by trawling. The invertebrate communities are also directly affected by trawling due to disturbance or mortality. Trawling can inhibit the growth of fish to their natural size as well. Trawl nets catch some species of fish so frequently they are unable to grow into adulthood before they are caught, which leads to truncated size distributions of those species. Trawl nets are also responsible for a shift in organisms of a site and trophic cascades, and cause an imbalance in the ecosystem. The studies conducted about trawls provides a plethora of evidence that confirm commercial trawl shrimp fisheries are unsustainable and cause detrimental harm to the ecosystem.
KEYWORDS
Commercial Fishery
Trawls
By-catch
Catch per Unit Effort
Ecosystem
INTRODUCTION
People all around the world love to eat shrimp and it serves as a staple in many cultural dishes. The high global demand for shrimp makes marine shrimp fisheries a very profitable and competitive market. The majority of all marine fisheries prioritize maximum profit by exploiting their target catch per unit effort. This drives competing shrimp fisheries to a variety of methods to outcompete each other. Commercial fisheries increase the amount of fishing effort by increasing their number of fishing vessels or by extending the duration of their fishing trips. There is advancement on fishing techniques and equipment to exploit the behavior and habitat preferences of target species. The greed of marine fisheries often leads to unsustainable fishing practices, a severe depletion of the target species, pollution and destruction of the marine habitat, and a negative effect on the ecosystem. In the case of shrimp fisheries, they maximize their catch per unit effort by using commercial fishing gear called a trawl. Trawling involves pulling a weighted, sock shaped net through a water column or on the sea floor in order to exploit the catch of a target species. Most commercial shrimp fisheries use beam trawls because they are specifically designed to target benthic species, like shrimp, that either bury or rest on the benthic sediment (Jennings and Kaiser, 1998). Beam trawls are comprised of a rigid beam held off the seabed by two beam shoes; the net headline is fixed at an open position and attached to the beam; the footrope is attached to the beam shoes (Jennings and Kaiser, 1998).

Beam trawl design.
Tickler chains are gear used with trawls because they are designed to penetrate the upper layers of the sediment in order to disturb the target species that are buried in the sediment (Jennings and Kaiser, 1998). The disturbance then forces the target species to swim up and into the path of the trawl. The other kind of trawl is called otter trawls. Otter trawls are comprised of two rectangular otter boards or doors, attached to the towing warps, which keep the mouth of the net open (Jennings and Kaiser, 1998). The heavy weight of the boards can penetrate the soft sediment up to 15 centimeters (Krost et al. 1990). Otter trawls also utilize invasive tickler chains that are attached between the otter boards (Harden-Jones and Scholes, 1974), or damaging rockhopper gear, a type of ground fishing gear that is hopped over rocky substrata (Jennings and Kaiser, 1998). Unfortunately, both categories of trawls are designed to prioritize maximum catch of anything in its path and do not consider the destructive effects it has on the ecosystem. Therefore, trawling is considered the world’s number one marine ecosystem damaging agent. Commercially fishing for shrimp with trawls is an unsustainable fishing practice and causes detrimental harm to the ecosystem.
BY-CATCH
Trawl inefficiency: amount of by-catch vs. target species
Trawls are one of the most unsustainable methods of fishing because trawls catch an outrageously high amount of by-catch in comparison to the amount of target shrimp or commercially profitable fish. Trawls run through the water column or on the sea floor and collect everything in its path, and the majority of organisms caught in trawls are useless by-catch. By-catch refers to marine species or organisms that are unintentionally caught while fishing for a target species. By-catch does not necessarily refer to the death of the inadvertently caught organisms; however, the by-catch of commercial fisheries often dies before it can be released back into the ocean. Fulanda (2003) determined that shrimp trawling in Ungwana Bay was a threat to marine resources due to trawls’ inefficiency. During a survey spanning for a total of 296 trawling hours for a seven-day period on three shrimp fishery vessels, shrimp only constituted of 13.7% of the total catch and 14.4% was commercially profitable fish of the total catch (Fulanda, 2003). This equates less than a third (28.1%) profitability of all the organisms caught within the trawl net. The rest of the catch within the trawl net (79.1%) is all by-catch that is often times discarded back into the ocean dead because these organisms are either non-commercial fish, juveniles, or benthic debris. The majority of the by-catch was due to non-commercial fish, such as Branchyura (crabs), leiognathidae (ponyfish), Apogonidae and Squillidae (Fulanda, 2003). The juveniles caught as by-catch were mostly undersized commercial fish (Fulanda, 2003). The high inefficiency of trawl nets is a serious threat to the future of the commercial fisheries. The commercial fisheries’ actions have a negative feedback on themselves, and if they continue to deplete their own resource, the fishery will not survive. Fortunately, there are some simple solutions to reduce the amount of by-catch caught by trawl nets. The high quantities of juveniles caught as by-catch could be easily avoided by decreasing the mesh size of the trawl nets and by avoiding trawling in shallow areas where juveniles primarily reside (Fulanda, 2003).
By-catch mortality rates
The by-catch organisms of commercial fisheries have varying mortality rates depending on the stress tolerance of the species. Meyer et al. (1999) found that mojarra were highly susceptible to mortality from the trawl nets and the mojarra encompassed 60% of the total catch in August (Meyer et al., 1999). This indicates that over 50% of the trawls’ catch possibly died as by-catch. The differing species mortality from trawling may have an influence on the species diversity and composition of that area with long term trawling (Meyer et al., 1999). The mortality rate of species exposed to trawl nets was also seen to have an inverse relationship with size. Fritz and Johnson (1987) found that a potential explanation for the differences in trawl mortality rate between small and large sized fish could be attributed to larger fish having a greater proportion of muscle tissue and larger energy stores than smaller fish; larger fish with these characteristics are able to avoid net contact during prolonged trawl tows. Therefore, littler, less strong fish are left susceptible to higher rates of stress and mortality from trawl nets. High mortality rates of small fish from trawl by-catch can have a cascading effect on the commercial fisheries. High mortality of small fish depletes an essential food source for juveniles and can reduce the stock of juvenile fish (Meyer et al., 1999). The decline of juveniles can reduce the reproductive potential of a target species and prevent that species from replenishing their stock. This, in turn, can hurt a commercial fishery because their target species’ stock is being exhausted.
By-catch dumping
Harris and Poiner (1990) assessed the quantity and composition of by-catch from commercial prawn trawling in Torres Strait, Australia over a 2-year period. They found that a wide range of marine organisms were caught as by-catch including cephalopods, crabs, rock lobsters, scallops, sharks, rays, snakes, turtles, and mostly teleost fish (Harris and Poiner, 1990). Apart from the small amount lobsters and squid, nearly all the by-catch (approximately 99%) was discarded dead (Harris and Poiner, 1990). Majority of the time, the by-catch, whether dead or alive, is dumped back into the ocean. The large amount of dead by-catch pollutes and contaminates the water, which can result in more organismal death in that area. Fulanda’s (2003) study also found that by-catch dumped back into the waters is an environmental and health hazard because of fouling and contamination of the waters and fish harvested by artisanal fishermen. This study found that a better use of by-catch is to “off-load” it to the artisanal fishing boats that have very low catches due to their low levels of fishing technology (Fulanda, 2003).
By-catch of infaunal species and effects
A study conducted by Jennings and Kaiser (1998) found that a trawl net’s by-catch of non-target infaunal species have drastically affected the benthic communities. Their study found that the by-catch of the infaunal bivalve, Arctica islandica, and the heart urchin, Echinocardium cordatum, by a beam trawl indicates that the tickler chains penetrated hard sandy substrate to at least a depth of 6 cm where these organisms primarily reside (Jennings and Kaiser, 1998). The invasive nature of trawls not only collects these benthic species as by-catch, but it also destroys the benthic habitat.
DESTRUCTION OF BENTHIC HABITAT
Trawling effects on different bottom types
Trawls have been designed to maximize their contact with the seabed in order to attempt to catch most amount of shrimp, however, this method demolishes the benthic habitat. The different types of bottom habitats have varying resilience to trawling. In order to understand how different bottom types respond to shrimp trawl fishing, Wells et al. (2008) conducted a study to identify the differences in community structure among sand, shell, and reef habitats in trawled and non-trawled areas in the Gulf of Mexico. They found differences in the habitat characteristics, and therefore, differences in the biotic community structure between trawled and non-trawled areas, which suggest that trawling may impact the benthic ecosystem (Wells et al., 2008). The patterns they observed from trawl fishing specifically indicated that more complex habitats, such as complex shell-rubble habitats, are more sensitive to the effects of fishing activities like trawling (Wells et al., 2008).
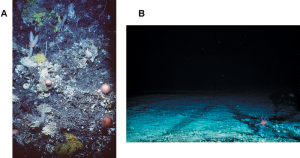
A. The coral community and seabed on an untrawled seamount; B. The exposed bedrock of a trawled seamount.
The destruction of complex habitats consequently reduces the amount of refuge for resident species, and can further result in a loss of species diversity and/or abundance of that trawled area.
Trawling varying effects of mud-bottom substrata
Multiple studies have indicated that the consequences of trawling are most apparent in relatively high relief, complex areas of seabed such as boulders, gravel, and rippled sand bottoms (Auster and Langton, 1999; Wells et al., 2008). However, trawling effects are still evident on mud substrate, and it can affect small-scale physical, chemical and biological habitats (Simpson and Watling 2006). Trawling on a mud-bottom habitat also may reduce the habitat structure by the removal or the death of the sediment dwelling organisms that increase the physical heterogeneity in soft sediments by burrowing and feeding (Simpson and Watling, 2006). Simpson and Watling (2006) further investigated the impacts of shrimp trawling on the mud-bottom habitat. They assessed the effects of trawl induced sediment disturbances on the habitat structure by the burrow density and the sediment porosity. Burrows increase the sediment surface area exposed to the overlying water column, are areas of chemical reactions with oxygen, dissolved metals and other elements in solution, and are sites of enhanced diagenesis and nutrient cycling (Aller, 1982). The reduction in burrow densities due to trawling is expected to impact the localized sediment elemental cycling and organic matter (Aller, 1994; Aller, 1982). Sediment porosity is and indicator of sediment grain size, arrangement, and the capability of the sediment to support animals (Simpson and Watling, 2006). Simpson and Watling (2006) found that trawling indeed had changed the porosity and sediment grain size to an extent, but it did not have a significant change to the porosity of the fishing grounds. In terms of burrow density, they found that the burrowing density was more abundant in trawled areas, which indicates that mud-bottom fishery grounds have a high recovery recolonization (Simpson and Watling, 2006). They concluded that in this area mud-bottom habitat could with stand trawl fishery efforts without a lasting impact on the overall habitat. However, Smith et al. (2000) also examined the effects of commercial trawl fisheries on a mud-bottom habitat in the eastern Mediterranean Sea and his study showed differing results. He found that there was a strong negative impact of trawling on the benthic macrofauna and megafauna, and a significant change in the sedimentary organic carbon, chlorophyll, and phaeopigments in trawled areas (Smith et al., 2000). The differences between the results of these two studies indicate that trawling has variable effects depending on the area being trawled and the bottom type of that area. Therefore, trawl-fishing grounds should be tested for resilience to trawl disturbance prior to committing a site to long term trawling.
Trawl destruction of seagrass beds
Shrimp trawling fisheries that profit off of the catch of live-bait shrimp target different fishing areas of high productivity. A live-bait shrimp trawl fisheries primarily target pink shrimp, Penaeus duorarum. These pink shrimp reside in beds of highly productive turtlegrass, Thalassia testudinum, therefore, live-bait shrimp fisheries run their trawls through these vital ecosystems in a quest for maximum catch per unit effort (Tabb and Kenny, 1969). Trawls have not been known to up root the seagrass beds because turtlegrass has an extensive root system; however, these trawls will damage and break off the turtlegrass leaves and collect them in the trawl net (Woodburn et al., 1957). The demolition of seagrass beds by trawls has the potential to change the composition of the local ecosystem by destroying a key habitat for many local species. Additionally, the trawl nets have a tendency to collect and redistribute the macroalgae and turtlegrass litter throughout different regions of the sea floor (Meyer et al., 1999). This redistribution can significantly reduce the habitat complexity of one area and enhance the complexity of another, which will make the benthic habitat unbalanced (Meyer et al., 1999). Consequently, altering the habitat can have a direct effect on the composition and abundance of the species that reside in that area.
Effects of resuspension of sediment due to trawling
Many benthic environments have a significant nepheloid layer, characterized as a near-bottom region of permanent sediment suspension (Pilskaln et al., 1998). High frequency commercial trawling may have significant effects on the resuspension fluxes of sediment and it may contribute to the maintenance of the nepheloid layer. Pilskaln et al. (1998) wanted to specifically see if the trawling in the Gulf of Mexico, where there is a thick and basin-wide nepheloid layer, increases the resuspension layer and the effects it has on the ecosystem. They observed the nepheloid layer after trawling disturbances of the infaunal organisms that characteristically live in the sediment. Polychaete worms are benthic, infaunal worms that do no swim far above the sediment water interface; however, polychaetes were found caught within the sediment traps deployed in various locations in the Gulf of Mexico (Pilskaln et al., 1998). These results suggest that the trawling fishing gear may be responsible for the artificial resuspension of the bottom sediments, and there by, either resuspending the worms from the sea floor into the nepheloid layer or discharging the worms from the net and bringing them to the surface near the trap site (Pilskaln et al., 1998). The resuspension of the sediments by trawling directly disturbs the polychaete worms from their natural habitat and it also has biogeochemical consequences. The majority of the nutrients in the continental shelf are from primary production from the sediment, which is derived from organic matter decay and nutrient remineralization (Pilskaln et al., 1998). Trawling effects the sediment nutrient fluxes in several ways. Trawling can bury the fresh, organic matter into subsurface horizons from its normal position at the sediment-water interface, which will shift organic matter decay from aerobic, eukaryotic populations towards anaerobic, prokaryotic metabolism (Mayer et al., 1991). Trawling can also physically enhance the upward flux of remineralized nutrients in the interstitial pore water of the sediment, which will result in the nutrients entering the water column in a large pulse rather than by the usual slower and steady mechanisms (Pilskaln et al., 1998). The abundance of nutrients at one time can have alterations in the rate and type of primary production (Pilskaln et al., 1998). These biogeochemical modifications to the sediment from trawling resuspension can have a cascade effect and also change the species composition of the area. Trawling changes an area chemically, physically, and biologically and these changes have corresponding effects on the organisms that reside in these areas.
ALTERATIONS IN THE INVERTEBRATE COMMUNITY
Invertebrate species response to direct trawling
Since trawls have significant effects on the benthic habitat, consequently, trawling alters the abundance and diversity of the benthic community.
Trawling an area can either directly affect the infaunal, invertebrate community by mortality and/or disturbance, or trawling can indirectly affect invertebrates by the destruction of their habitat and/or chemical changes to the sediment. Simpson and Watling (2006) found that there are clear disturbances in the macrofaunal community structure in areas of recently shrimp-trawled areas, where as, there was no detection of disturbance in areas of older (minimum of a year prior) trawling activity. Specifically, trawling-sensitive taxa, like bivalve families Nuculidae and Nuculanidae, were more abundant in untrawled areas because these species could not tolerate the damaging effects of trawls (Simpson and Watling, 2006). Many studies, including Simpson and Watling (2006), have observed trawling affecting invertebrate species differently depending on their life histories. Invertebrate species of large, long-lived animals living near the surface of the sediment are predicted to be more sensitive to trawling disturbance, where as, small, fast growing, regenerating and mobile animals are expected to be more dominant in trawled areas (De Groot, 1984; Rumohr and Krost, 1991; Hall, 1994; Kaiser 1998; Thrush et al., 1998). Unfortunately, this sensitivity corresponds to their mortality rate to trawls as well. The mortality rates of infaunal organisms due to trawling depend on certain life history characteristics, such as body size and depth distribution within the sediment (Duplisea et al., 2002). Fortunately, the macrofaunal communities on mud-bottom trawling grounds have fast recovery rates due to their high reproductive output (Simpson and Watling, 2006). The invertebrates of mud-bottom trawling sites, in comparison to more sensitive species in other habitats, are capable of withstanding the consequences of trawling if there is an adequate amount of time given for recovery between trawls. However, over harvesting or long-term trawling of a centralized location, even a tolerant habitat like a mud-bottom, could result in a semi-permanent degradation of the invertebrate abundance and diversity (Jones, 1992; Auster et al., 1996, Gray, 1997, Jennings and Kaiser, 1998).
Hansson et al. (2000) sought to study the effects of trawling on assemblages of macro fauna in Gullmarsfijorden, Sweden. The study found that there was an overall appearance of a negative trend in abundance of macrofauna within the fjord. Explicitly, the negative effects of trawling declined the abundance of echinoderms, especially the ophiurids (brittle starts) (Hansson et al., 2000). Hansson et al. (2000) found that the ophiurids’ population decreased by an average of 30% at trawled sites in comparison to the untrawled sites. These results are somewhat surprising because ophiuroids have the ability to regenerate lost body parts and it would have been assumed that they would be resistant to trawling (Lindley et al., 1995; Kaiser and Spencer, 1996; Tuck et al., 1998). There is a possibility that the decline in abundance of ophiuroids is due to responses to oxygen stress (Hansson et al., 2000). Ophiuroids may have migrated from protected sediment to a more exposed position on the sediment surface as a response to sub-lethal hypoxia (Rosenberg et al., 1991) induced by the absence of water renewal in the deep parts of the fjord in 1997 (Hansson et al., 2000). Once the Ophiuroids were exposed to the surface of the sediment, they were also exposed to the shrimp trawl nets that either directly destroyed their bodies beyond regeneration or caught them as by-catch.
Invertebrate species response to indirect trawling
Some Invertebrate species are sensitive to alterations in their environment and can be indirectly affected by trawling that destroys their habitat. In looking at the invertebrate community structure after trawling, Wells et al. (2008) study indicated that some invertebrate species such as two brittle stars (O. appressum and O. elegans), a sea star (L. clathrata), and urchin (A. punctulata), a hairy sponge crab (D. antillensis), the shortfinger neck crab (P. sidneyi), and brown rock shrimp (Sicyonia brevirostris) are more abundance in structurally complex, non-trawled areas. Essential complex habitats provides shelter and protection for these invertebrates, and without these structures, these invertebrates are vulnerable and susceptible to predation. Therefore, these species are more sensitive to trawling because it destroys their complex habitats necessary for their survival. The shrimp trawling has variable direct and indirect effects on the invertebrate community depending on the species and the bottom type sediment of a fishing ground; however, the negative impacts of trawling can create a bottom up cascade from the invertebrates to other species of the ecosystem.
DISTRUPTIONS IN THE FOOD WEB AND ECOSYSTEM
Trawls destruction of habitats drive an ecosystem shift
The food web and ecosystem are severely disrupted by the destructive method of trawling and the shrimp commercial fisheries’ high consumption of target species and by-catch. The loss in the structural benthic habitat can result in a shift of residing marine organisms. Wells et al. (2008) found that with the loss of the structural epibenthic community due to trawling resulted in a shift from snappers (Lutjanidae) and emperors (Lethrinidae) towards an ecosystem dominated by lizardfish (Synodontidae) and bream (Nemipteridae). In general, trawled areas become inhabited by species with similar life characteristics, including: small size, short life spans, high mortality, and rapid biomass turnover (DeVries and Chittenden, 1982; Geoghegan and Chittenden, 1982; Murphy and Chittenden, 1991; McEachran and Fechhelm, 1998). Species including the longspine porgy, silver seatrout, large-scale lizardfish, and gulf butterfish are all species that successfully inhabit trawled areas because their life history characteristics will allow them to recover swiftly after the damage (Wells, Cowan, and Patterson, 2008). The destruction to habitats caused by trawls has an influence on the entire ecosystem composition and potentially reduces species diversity.
Trawling triggers truncated size distributions
Some fish species in trawling areas have been seen to have truncated size distributions and reduced median size. In the Gulf of Mexico, the species that showed truncated size distributions over trawled areas were the same species that were the most abundant caught as by-catch in the shrimp trawl fishery (Wells et al., 2008; Chittenden and McEachran, 1976). Similarly, other studies have found that trawling and dredging is the cause of a decrease in biomass and average size of demersal fish and invertebrate fauna (Bianchi et al., 2000; Zwanenburg, 2000, Duplisea et al., 2002). The observations of truncated size distributions of local fish indicates that a species is caught at such a high frequency that they are unable to grow into adulthood before they are caught and captured. This can severely affect the population, abundance, and health of a species.
Manipulation of the ecosystem into a top-down trophic cascade
Trawling also has the ability to manipulate the ecosystem in ways to actually produce more target shrimp by reducing the amount of predators. Salcido-Guevara et al. (2012) discovered that shrimp trawling in La Paz Bay had positive impacts on the shrimp populations because the by-catch from the trawl nets actually reduced the amount of shrimp predators. This study suggested that predator removal had a strong positive impact on the shrimp’s population than does the negative impact of fishing the shrimp (Salcido-Guevara et al., 2012). These results are alarming because the human manipulation of an ecosystem does not always end with the results that are desired or expected. By purposefully decreasing the amount of shrimp predators for their benefit, the fisheries are creating a top-down trophic cascade. A top-down trophic cascade suggests that the apex predators’ populations have the power to alter the rest of the food web; for example, if there is a reduction or depletion of predators, there will be a rise in prey’s population. This trophic cascade continues down the food web and will eventually either deplete or alter the diversity of the primary producers. Trophic cascades can throw the ecosystem food web out of balance, causing expansive negative effects that were not initially considered. Nonetheless, under heavy shrimp fishing pressure, both shrimp and the predator’s biomasses will sharply decline as expected (Salcido-Guevara et al., 2012).
DISCUSSION
Shrimp fisheries current trawling methods can have extensive negative impacts on the habitat structure, the benthic invertebrate community, the shrimp population, other local species abundance and diversity, and the overall balance of the ecosystem. The shrimp catch of the commercial trawl fisheries do not out weigh the externality that trawls exert on the ecosystem. With long term and frequent trawling of an area, eventually, benthic habitat will be destroyed, the species diversity and abundance will decrease, and in turn, the target species will also decline. Therefore, there is a negative feedback on the shrimp fishery when utilizing the current design of trawls in high frequency. The implications of these findings confirm that changes need to be made before the destruction of trawling is irreversible. There are some potential solutions to reduce the damaging impacts of trawling. The avoidance trawls is the most effective way to prevent damage; therefore, educating people about the unsustainability of commercial shrimp fisheries and encouraging people to reduce their intake of shrimp can have a large impact on the reduction of damage by trawling. If there is a decline in the demand of shrimp, then the shrimp fisheries will reduce their trawling efforts. Another potential solution is providing alternatives to trawling. Although it’s a less practical solution, self-catching shrimp with a net is encouraged because it is the least invasive to the environment. By employing shrimp catching as a sport or as an ecotourism attraction, people can catch their own dinner and have fun doing it. However, the problem with this is that most people do not live in an area where they can catch their own shrimp so readily.
Commercial shrimp fisheries can also implement their own modifications to their current trawling methods in order to help reduce damage. By limiting the amount of time per trawl tow and the trawl frequency of an area, the bottom habitat and local organisms will be given a chance to recover. Trawling time constraints will prevent a site from being over exploited beyond recovery. Shrimp trawls can also reduce negative impact by modifying the area and the depth at which they tow. Shrimp trawling is seen to have the least destructive effects on certain mud-bottom substrata (Simpson and Watling, 2006). Damage to complex structures and essential habitats can be avoided if commercial shrimp fisheries localized their trawling to a more stress tolerant area, such as mud-bottom habitats. Fulanda (2003) found that trawling in shallow areas increases their by-catch of juveniles. Therefore, regulations set for a minimum trawl depth will prevent trawls from being towed in too shallow areas where juveniles primarily reside. Modifications to the net design can further improve the reduction of by-catch. Decreasing the mesh of the trawl net can reduce the amount of small fish by-catch and juvenile by-catch (Fulanda, 2003). There are a variety of potential solutions to the damaging effects of trawl nets, but first, the attitude of the commercial shrimp fisheries need to change. Commercial shrimp fisheries need to stop prioritizing their maximum profit for “right now,” and instead, start considering the effects their fishing practices have on the ecosystem and the future of their fishery. With a changed mindset, initiatives for sustainable fishing will be prioritized and shrimp fishing will pose less damaging consequences on the ecosystem.
REFERENCES
Aller RC (1982) The effects of macrobenthos on chemical properties of marine sediment and overlying water. Animal Sediment Relations, pp. 53-102. Ed. by P. L. McCall, M. Tevez. Plenum Press, New York.
Aller RC (1994) Bioturbation and remineralization of sedimentary organic matter effects of redox oscillation. Chemical Geology, 114: 331-345
Auster PJ, Langton R (1999) The effects of fishing on fish habitat. American Fisheries Society Symposium 22: 150-187
Auster PJ, Malatesta RJ, Langton RW, Watling L, Valentine PC, Donaldson CL, Langton EW, Shepard AN, Babb IG (1996) The impacts of mobile fishing gear on seafloor habitats in the Gulf of Maine (Northwest Atlantic): Implications for conservation of fish populations. Rev Fish Sci 4: 185-202
Bianchi G, Gislason H, Graham K, Hill L, Jin X, Koranteng K, Manickchand-Heileman S. et al. (2000) Impact of fishing on size composition and diversity of demersal fish communities. ICES Journal of Marine Science 57: 558-571
Chittenden ME, McEachran JK (1976) Composition, ecology, and dynamics of demersal fish communities on the Northwestern Gulf of Mexico continental shelf, with a similar synopsis for the entire Gulf. Texas A&M University Sea Grant Publication 76-208, College Station, TX.
De Groot SJ (1984) The impact of bottom trawling on benthic fauna fo the North Sea. Ocean Manage 9: 177-190.
DeVries DA, Chittenden ME (1982) Spawning, age determination, longevity, and mortality of the silver seatrout, Cynoscion nothus, in the Gulf of Mexico. Fishery Bulletin US 80: 487-500
Duplisea DE, Jennings S, Warr KJ, Dinmore TA (2002) A size-based model of the impacts of bottom trawling on benthic community structure. Canadian Journal of Fisheries and Aquatic Sciences 59: 1785-1795
Fritz KR, Johnson DL (1987) Survival of freshwater drums released from Lake Erie commercial shore seines. N. Am. J. Fish. Manage. 7: 293-298.
Fulanda B (2003) Shrimp Trawling in Ungwana Bay A Threat to Fishery Resources. African Studies Centre: 233- 242
Geoghegan P, Chittenden ME (1982) Reproduction, movements, and population dynamics of the longspine porgy, Stenotomus caprinus. Fishery Bulletin US 80: 523-540
Gray JS (1997) Marine biodiversity: patterns, threats and conservation needs. Biodivers Conserv 6: 153-175
Hall SJ (1994) Physical disturbance and marine benthic communities: life in unconsolidated sediments. Oceanogr Mar Biol Annu Rev 32: 179-239.
Hansson M, Lindegarth M, Valentinsson D, Ulmestrand M (2000) Effects of Shrimp-Trawling on abundance of benthic macrofauna in Gullmarsfjorden, Sweden. Mar Ecol Prog Ser 198: 191-201
Harden-Jones FR, Scholes P (1974) The effect of door-to-door tickler chain on the catch-rate of plaice (Pleuronectes platessa L.) taken by an otter trawl. Journal du Conseil International pour I’exploration de la Mer 35: 210-212.
Harris AN, Poiner IR (1990) By-catch of the prawn fishery of Torres Strait; composition and partitioning of the discards into components that float or sink. Mar Freshwater Res 41: 53-64
Jennings S, Kaiser MJ (1998) The effects of fishing on marine ecosystems. Adv Mar Biol 14: 201-352
Jones JB (1992) Environmental impact of trawling on the seabed: a review. NZ J Mar Freshw Res 26: 59-67
Kaiser MJ, Spencer BE (1996) The effects of beam-trawl disturbance on infaunal communities in different habitats. J Anim Ecol 65: 348-358.
Kaiser MJ (1998) Significance of bottom-fishing disturbance. Conserve Biol 12: 1230-1235.
Krost P, Bernhard M, Werner F, Hukriede W (1990) Otter trawl tracks in Kiel Bay (Western Baltic) mapped by side-scan sonar. Meeresforschung 32: 344-353.
Lindley JA, Gamble JC, Hunt HG (1995) A change in the zooplankton of the central North Sea (55O to 58O N): a possible consequence of changes in the benthos. Mar Ecol Prog Ser 199: 299-303.
Martinet V, Blanchard F (2009) Fishery externalities and biodiversity: Trade-offs between the viability of shrimp trawling and the conservation of Frigatebirds in French Guiana. Ecological Economics 68: 2960-2968
Mayer LM, Schick DF, Findlay R, Rice DL (1991) Effects of commercial dragging on sedimentary organic matter. Marine Environmental Research 31: 249-261
McEachran JD, Fechhelm JK (1998) Fishes of the Gulf of Mexico. University of Texas Press, Austin, TX.
Meyer DL, Fonseca MS, Murphey PL, McMichael RH Jr, Byerly MM, LaCroix MW, Whitfield PE, Thayer GW (1999) Effects of live-bait shrimp trawling on seagrass beds and fish bycatch in Tampa Bay, Florida. Fish Bull 97: 193-199
Murphy MD, Chittenden ME (1991) Reproduction, age and growth, and movements of the gulf butterfish Peprilus burti. Fishery Bulletin US 89: 101-116
Pilskaln CH, Churchill JH, Mayer LM (1998) Resuspension of sediment by bottom trawling in the Gulf of Maine and potential geochemical consequences. Conservation Biology 12: 1223-1229
Rooper CN, Wilkins ME, Rose CS, Coon C (2011) Modeling the impacts of bottom trawling and the subsequent recovery rates of sponges and corals in the Aleutian Islands, Alaska. Continental Shelf Research 31: 1827-1834
Rosenberg R, Hellman B, Johnsson B (1991) Hypoxic tolerance of marine benthic fauna. Mar Ecol Prog Ser 79: 127-131.
Rumohr H, Krost P (1991) Experimental evidence of damage to benthos by bottom trawling with special reference to Arctica islandica. Meeresforsch Rep Mar Res 33: 340-345.
Salcido-Guevara LA, Monte-Luna P, Arreguín-Sánchez F, Cruz-Escalona VH (2012) Potential ecosystem level effects of a shrimp trawling fishery in La Paz Bay, Mexico. Open Journal of Marine Science 2: 85-89
Simpson AW, Watling L (2006) An investigation of the cumulative impacts of shrimp trawling on mud-bottom fishing grounds in the Gulf of Maine: effects on habitat and macrofaunal community structure. ICES Journal of Marine Science 63: 1616-1630
Smith CJ, Papadopoulou KN, Diliberto S (2000) Impact of otter trawling on and eastern Mediterranean commercial trawl fishing ground. ICES Journal of Marine Science, 57: 1340-1351
Tabb DC, Kenny N (1969) A brief history of Florida’s live bait shrimp fishery with description of fishing gear and methods. FAO fish Rep. 57: 1119-1134
Thrush SF, Hewitt JE, Cummings VJ, Dayton PK (1995) The impact of habitat disturbance by scallop dredging on marine benthic communities: what can be predicted from the results of experiments? Mar Ecol Prog Ser 129: 141-150.
Tuck ID, Hall SJ, Robertson MR, Armstrong E, Basford DJ (1998) Effects of physical trawling disturbance in a previously unfished sheltered Scottish sea loch. Mar Ecol Prog Ser 162: 227-242.
Van Dolah RF, Wendt PH, Levisen MV (1991) A study of the effects of shrimp trawling on benthic communities in two South Carolina sounds. Fisheries Research 12: 139-156
Warnken KW, Gill GA, Dellapenna TM, Lehman RD, Harper DE, Allison MA (2003) The effects of shrimp trawling on sediment oxygen consumption and the fluxes of trace metals and nutrients from estuarine sediments. Estuarine, Coastal and Shelf Science 57: 25-42
Wells RJD, Cowan JH Jr, Patterson WF III (2008) Habitat use and the effect of shrimp trawling on fish and invertebrate communities over the northern Gulf of Mexico continental shelf. ICES Journal of Marine Science 65: 1610-1619
Woodburn KD, Eddred B, Clark E, Hutton RF, Ingle RM (1957) The live bait shrimp industry of the west coast of Florida. Florida State Board Conserv. Tech. Ser. 21: 3-33
Zwanenburg KCT (2000) The effects of fishing on demersal fish communities of the Scotian Shelf. ICES Journal of Marine Science 57: 503-509