by Alice Schreiber, RJD intern
The rapid increase of world population and the large number of already depleted fish stocks pose a significant problem for fisheries currently, and will continue to do so in the future. The growth of responsible and sustainable aquaculture facilities can help to alleviate the strain the human population has on the natural environment while providing reasonably priced seafood to people in developing and developed nations around the world. Unfortunately, there are still some issues facing aquaculture today, the most contentious of which associated with sustainability and waste production. Integrated multi-trophic aquaculture combines traditional fish farming with another species at a different trophic level, usually an extractive species that can get its nutrients from the sunlight or water.
In recent years, aquaculture has begun to be a major player in providing food security throughout the world. In 2012, global aquaculture production reached an all-time high of 90.4 million tons; China alone attributed 43.5 million tons of fish for food and 13.5 million tons of aquatic algae that year. Aquaculture growth has been relatively faster in Africa, Asia, Latin America, and the Caribbean; the same regions where population growth has been increasing. Along with the increase in production, the interest in integrated multi-trophic aquaculture is also rising. These systems, abbreviated as IMTA, combine fed aquaculture species with inorganic extractive species, such as seaweeds, cultivated in proximity. Figure one illustrates a typical IMTA:
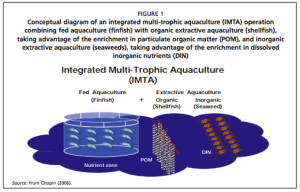
Many aquaculture systems tend to require very large amounts of inputs and produce massive amounts of waste. The goal is to essentially close the loop, or make the inputs and outputs as low as possible. “Rather than let huge concentrations of fish manure from, say, salmon cages foul coastal waters, you place shellfish, which filter and are nourished by the manure, slightly downstream from your salmon cages; and then seaweed further downstream still, which takes up remaining nutrients from the manure” (Greenaway, 2009). Another issue with traditional aquaculture is the amount of fish used to make fish feed. However, seaweed can be a source of protein and other ingredients without competing with terrestrial plants and causing price increases. This also could help reduce the need for farmland, irrigation, and fertilizer (Chopin, 2012). Shellfish and seaweed can take the excess nutrients and utilize it, converting it into biomass. Dr. Thierry Chopin states that the solution to nitrification is not dilution, but conversion within an eco-system based management perspective (Chopin, 2008).
The waste generated from intensive aquaculture systems needs to be treated and in the past solutions have largely focused on reducing particle load or leaving the dissolved nutrients untreated. Treating effluents is expensive and requires a high degree of technology, so releasing the untreated water is unfortunately a way to cut costs. Macroalgae uses sunlight to build biomass, while assimilating dissolved inorganic nutrients removed from the water. If properly cultured, the seaweed can utilize pollutant nutrients as their food and energy source, clean the water, and be harvested as commercial crops with very little added cost to the producer. Recycling of waste nutrients by algae and filter-feeders is the most economical way to improve aquaculture sustainability.
Water quality deterioration as a result of excess nutrients is a key concern in aquaculture systems. Seaweed can be cultivated in the same pond as fish or adjacent to fish cages. The plants absorb particulate organics and dissolved nutrients that would otherwise enter the environment. In a recirculating aquaculture system (RAS), where water is filtered and reused in the tank, fish are even more at risk from high nutrient levels. Seaweed has the potential to improve culture environment by preventing the deterioration of water quality from waste and particulates. Seaweed assimilates the fish-excreted ammonia, phosphate and CO2, converting them into valuable biomass. With this treatment, water can recirculate back to the fish ponds or be discharged without endangering the environment (Chopin et al., 2001 and Neori et al., 2004). CO2 is released by fish through respiration, reacts with water, and forms carbonic acid (H2CO2), which leads to a lower pH (Wurts, 1992). When pH lowers, acidity increases, which can easily prove fatal for fish. Excessive phosphorus can cause increased plant an algal growth, resulting in anoxia (low or no oxygen), increased turbidity, effects on fish growth, and toxic cyanobacterial blooms (Guo et al., 2003). Unionized ammonium is particularly harmful as it can pass through the gills into fish, convert back into the ionized for, and cause cellular damage.

The advantages of using seaweeds as the biofilter component of the IMTA systems are now becoming widely accepted (Ridler et al., 2007). Seaweed can greatly help mitigate eutrophication in polluted areas and reduce harmful fish byproducts. Macroalgae (seaweed) actually sequesters the nutrients out of the water, and then the clean and oxygen-rich effluent of a seaweed biofilter can be recirculated back to the fishponds or discharged safely. In seaweed-based IMTA systems, TAN (total ammonia N, NH3+NH4) and the other excess nutrients from the fed finfish/shrimp culture are taken up by seaweed.
Aquaculture is not a perfect industry as of yet. Macroalgae, or seaweed can provide multiple benefits when used in IMTA. Seaweed has potential as food, cosmetics, biofuel, agrichemicals, fishmeal, and is able to function as a biofilter for the aquaculture systems. The IMTA approach to fish farming is proving to be very beneficial economically as well as for the environment. Not only can seaweed help to maintain clean water quality, it can be used to clean up areas with excess nutrients or harmful waste products like ammonium and CO2. This will likely become the new standard for aquaculture as demand continues to rise and the need for environmentally safe practices increases.
Chopin, T. “Integrated Multi-Trophic Aquaculture (IMTA) – the right move for sustainability?” in Inshore Ireland 4 (3): 20, June 2008.
Chopin, T. “Seaweed aquaculture provides diversified products, key ecosystem functions. Part II. Recent evolution of seaweed industry.” Global Aquaculture Advocate 15 (4): 24-27, July-August 2012.
Greenaway, T. “Closing the aquaculture loop.” Culinate, 5 February 2009.
Guo, Longgen, and Zhongjie Li. “Effects of nitrogen and phosphorus from fish cage-culture on the communities of a shallow lake in middle Yangtze River basin of China.” Aquaculture 226.1 (2003): 201-212.
Neori, Amir, et al. “Integrated aquaculture: rationale, evolution and state of the art emphasizing seaweed biofiltration in modern mariculture.” Aquaculture 231.1 (2004): 361-391.47–131.
Ridler, N., et al. “Integrated Multi− Trophic Aquaculture (IMTA): A Potential Strategic Choice for Farmers.” Aquaculture Economics & Management 11.1 (2007): 99-110.
Wurts, William A., and Robert M. Durborow. Interactions of pH, carbon dioxide, alkalinity and hardness in fish ponds. Stoneville,, Mississippi: Southern Regional Aquaculture Center, 1992.