Modeling for Management: Predicting Ideal Conditions for Seagrass Habitat
By Emily Rose Nelson, RJD Intern
Seagrasses are an essential part of the marine ecosystem. They provide food, habitat, and safe nursery areas to a wide range of species. Seagrasses help to stabilize the sea floor during intense currents and storms, filter nutrients coming from land-based runoff, increase water clarity by trapping sediments, generate oxygen, and store excess carbon. Unfortunately, seagrass area is in significant decline around the world largely due to cumulative impacts of human activities such as coastal development, increasing pollution, and reckless boating. It is of utter importance that conservation and restoration efforts are put into place in order to protect seagrasses the ecosystem services they provide.
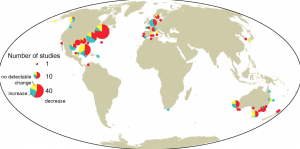
Map showing changing in seagrass area since 1879 at 205 sites along coastlines worldwide (Waycott et al., 2009).
To date, restoration efforts have been largely unsuccessful. In order to effectively reestablish seagrass area, knowledge of the environmental factors that impact seagrass is necessary. Presence requires a number of environmental conditions including light availability, wave height, and sediment characteristics to be satisfied. Knowing this, Adams et al. have created a mathematical model to link environmental conditions to the presence or absence of seagrass.
Moreton Bay, Australia is subtropical shallow coastal embayment. There is decades of extensive data on seagrass cover and environmental conditions available for this area, making it the perfect location to use to develop this model. The model takes into account three of the most important factors in the success of seagrass: light levels (represented by mean annual benthic light availability), physical wave conditions (represented by significant wave height), and geological sediment conditions (represented by mud concentration). Looking at previous data and performing a number of mathematical manipulations established limitations for each of the three environmental factors. Seagrass will only be present when the following conditions are satisfied: annual benthic light availability is greater than 9molm-2d-1, mean significant wave height is less than 0.6m, and sediment mud concentration is less than 50%. The study area was then divided into 100m by 100m cells and the presence or absence of seagrass was tested for each cell using the mathematical model.
Application of the model to Moreton Bay, Australia provided promising results. When compared to a real seagrass map from 2004, the model correctly predicted seagrass presence or absences at 85% of the cells. The model did even better when compared to a real seagrass map from 2011, correctly predicting 88% of the cells. Further, it is possible that some of the incorrect cells, in particular false positives, correspond to areas of opportunity for future seagrass growth.
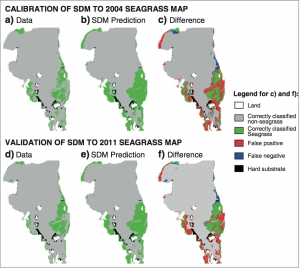
Real seagrass observational data compared to predictions using the model developed by Adams et al. for 2004 and 2011. a, b, and c are based on seagrass observed in 2004 and d, e, and f are based on seagrass observed in 2011. a/d show the observed seagrass data, b/e show the predicted seagrass using the model, and c/f show the difference between the real observations and the model predictions.
The success of the model created by Adams et al. provides hope for combining continual monitoring with modeling as a method to determine actions needed for conservation and restoration of seagrass beds on a local level. The limiting environmental factors differ among locations and therefore different actions are needed to improve chances of seagrass survival; if the model predicts absence of seagrass at a particular spot there is an environmental reason for that. If the area does not have enough sunlight efforts should be made to improve water clarity, and thus allow more light through. If the area has intense wave conditions, actions can be put in place to weaken the physical effects of waves. Knowing a specific reason why seagrass is absent in a particular area makes it easier for policy makers to successfully manage the area.
However, there are fallbacks to this model. For one, there are several other environmental variables that effect seagrass that are not taken into account. The model also does not account for interactions between seagrass abundance and the environmental conditions. It is also important to consider that management decisions, such as adding a break wall to minimize wave action, will likely affect other environmental factors indirectly. Despite some issues with this model, it does provide a start. Further work, such as adding additional environmental variables to the model, has the potential to make modeling an effective tool for restoration and conservation of seagrasses.
Reference:
Adams, M. P., Saunders , M. I., Maxwell, P. S., Tuazon , D., Roelfsema, C. M., Callaghan, D. P., et al. (2015). Prioritizing localized management actions for seagrass conservation and restoration using a species distribution model. Aquatic Conservation: Marine and Freshwater Ecosystems.
Waycott, M., Duarte, C.M., Carruthers, T.J.B., Orth, R.J., Dennison, W.C., Olyarnik, S., et al. (2009). Accelerating loss of seagrasses across the globe threatens coastal ecosystems. Proceedings of the National Academy of Sciences of the Unites States of America.