Disrupting a Biological Clock: Ticking Away Towards Further Environmental Contamination
By Casey Dresbach, SRC Intern
In an anthropogenic epoch, where industrial growth has become evermore prolific, threats of disturbance continue to change the environment. Some of these disturbances include habitat destruction and pollution, both of which threaten biodiversity and healthy ecosystems worldwide. (Palumbi, 2001; Dudgeon et al., 2006; Sih, Ferrari, & Harris, 2011). Fortunately, organisms may be able to adapt to changes in their environment to cope with this territorial renovation. These adaptations allow for species to persist under conditions that are polluted by destructive contaminants. However, such adaptation can also be associated with unforeseen tradeoffs that can actually pose consequential threats to a species’ existence. Reduced reproduction, smaller body sizes or increased susceptibility to other environmental stressors are just a few of these repercussions. (Ghazy, Habashy, Kossa, & Mohammady, 2009; Jansen, Stoks, Coors, van Doorslaer, & de Meester, 2011; Latta, Weider, Colbourne, & Pfrender, 2012)
Salinization of certain freshwater environments is, unfortunately, a frequent modern trend. Inherent from sources such as seawater intrusion, mining, and road salt, such disturbance has already forced many organisms into adaptive measures. Road salt, also known as NaCl, is commonly used in northern climates to clear streets and sidewalks of snow; it works as a de-icing aid (Learn, 2017). Continuous research efforts are aimed at the consequences of where the salt is ultimately absorbed in the seasons to follow once the climate warms up again. Road salt does not simply dissolve into the air, but instead splits into sodium and chloride ions that are then absorbed by roadside plants, and ultimately find their way into freshwater environments. (Godwin, Hafner, & Buff, 2002).
Zooplankton are especially important in freshwater ecosystems because they are both primary feeders on phytoplankton and a food source for other small fish. When looked at on a food chain, it is evident that they are essential to stabilizing an ecosystem. (See Figure 1) Ecosystem services are the material or energy outputs of an ecosystem that can benefit humans and other life. Some of these services may include food, water, and other resources (Teeb Web, n.d.).
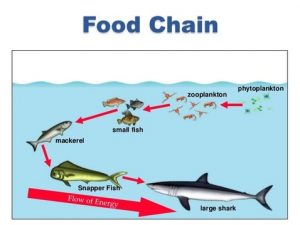
Figure 1. Oceanic ecosystem showing zooplankton as primary feeders on phytoplankton, showing the species as both the predator and the prey. (Belle Isle Conservancy, 2017).
In a recent study, an important species of zooplankton, Daphnia pulex was carefully studied within the context of their increasing tolerance to road salt, a common contaminant. As their freshwater habitats become saltier, Daphnia, among many other species, are forced to adapt at the expense of some of their regulatory genes. Researchers found that this salinization disturbance causes a major disruption in their circadian rhythm, a 24 hour cycle in the physiological process of living organisms, including plants, animals, fungi and cyanobacteria (Science Daily, 2017). By looking at a certain regulatory gene called period, they found suppressed, or as they called it “ablated”, levels of the gene’s oscillation. Unlike period, several other genes showed higher levels of expression indicating staggering increases in adaptation to high levels of salinity in these freshwater habitats. So unfortunately, while several other genes were adapting to new standards, the putative clock gene, period, was suppressed, meaning the circadian rhythm of the Daphnia species was minimized.
Daphnia pulex is an example of a species that is highly capable of adapting – evident in several of their genes, including, clock, actin, and the gene that regulates Na+/K+-ATPase. The species is also a prime example of overcompensation; capable of adaptation yes, but at the expense of an important regulatory gene. As mentioned earlier, a disturbance such as habitat destruction can affect reproduction, growth, longevity, and other behaviors such as diel vertical migration (DVM), a daily mass movement of zooplankton to the sea surface (Coldsnow, Relyea, & Hurley, 2017). In general it describes migrations upward at dusk and downward around dawn. During this migration, organisms migrate towards the surface at night to feed and then back down the water column during the day to minimize predation. By remaining at depth during the day in dimly lit water and ascending at night, they minimize their vulnerability to visual predators. The period gene is used as an external cue as to when zooplankton should migrate to feed. If that gene is suppressed, their circadian rhythms will be offset. A decline in Daphnia results in an increase in the trophic level above, as well as a loss of ecosystem services (See Figure 2).
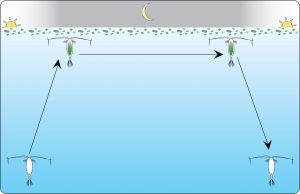
Figure 2. Zooplankton’s 24-hour migration cycle known as Diel Vertical Migration (DVM). (Planktoneer, 2008).
This study is an important initiative in a world of increasing anthropogenic disturbances. Urbanization will continue to change the natural world and, unfortunately, so will its detrimental consequences. More research should be done to investigate further implications in regards to environmental changes.
References
Belle Isle Conservancy. (2017). Food Chains. Retrieved from Detroit Aquarium: (https://detroitaquarium.weebly.com/uploads/2/5/7/5/25755066/editor/ocean-ecosystem-in-bali-8-638.jpg?1489083980)
Coldsnow, K. D., Relyea, R. A., & Hurley, J. M. (2017, October 28). Evolution to environmental contamination ablates the circadian clock of an aquatic sentinel species. (Wiley, Producer) Retrieved from Wiley Ecology and Evolution: http://onlinelibrary.wiley.com/doi/10.1002/ece3.3490/epdf
Godwin, K. S., Hafner, S. D., & Buff, M. F. (2002, December 5). Long-term trends in sodium and chloride in the Mohawk River, New York: the effect of fifty years of road-salt application. Environmental Pollution.
Learn, J. R. (2017, May 26). The Hidden Dangers of Road Salt. Retrieved from Smithsonian : https://www.smithsonianmag.com/science-nature/road-salt-can-disrupt-ecosystems-and-endanger-humans-180963393/
Planktoneer. (2008). Forays and Foraging in Marine Zooplankton. Cambridge, MD, USA.
Science Daily. (2017). Circadian Rhythm – Reference Terms. Retrieved November 10, 2017, from Science Daily: https://www.sciencedaily.com/terms/circadian_rhythm.htm
Teeb Web. (n.d.). Ecosystem Services. Retrieved November 10, 2017, from The Economics of Ecosystems & Biodiversity (TEEB): http://www.teebweb.org/resources/ecosystem-services/