by Becca Shelton, RJD Intern
Ever wonder why you have to limit certain types of seafood? Or why you should not eat certain types of seafood? Today, I will help answer these questions with scientific research. As an avid ocean enthusiast and lover of sustainable seafood, it is crucial to understand what is floating around in the water with marine life. And these potential health issues are not just restricted to humans! Marine fauna are also negatively affected by bioaccumulation and biomagnification, especially apex predators like sharks, seals/sea lions and killer whales. This post is going to briefly touch some of the different ways bioaccumulation and biomagnification affect marine apex predators and us.
The easiest way to understand how bioaccumulation and biomagnification work is to use them in a food chain scenario. Bioaccumulation begins at the first level of a food chain where there is an increase in the concentration of a pollutant from the environment to the first consumer (i.e. pollutants to plankton to filter feeder). It can also refer to the amount of toxins in individual animals because as a top predator consumes multiple, contaminated food sources, it will “accumulate” more toxins. Biomagnification occurs when the concentration of a pollutant increases from one link in the food chain to another (i.e. polluted fish will contaminate the next consumer and continues up a tropic food web as each level consumes another) and will result in the top predator containing the highest concentration levels. In order for biomagnification to occur, a pollutant must be long-lived, mobile, soluble on fats and biologically active (http://www.marietta.edu/~biol/102/2bioma95.html). Examples of these types of pollutants include DDT, polycholorinated biphenyls (PCBs), heavy metals (mercury, lead, chromium, etc.) and β-Methylamino-L-alanine (BMAA). In high enough concentrations, these toxins can lead to serious health issues for marine fauna and humans.
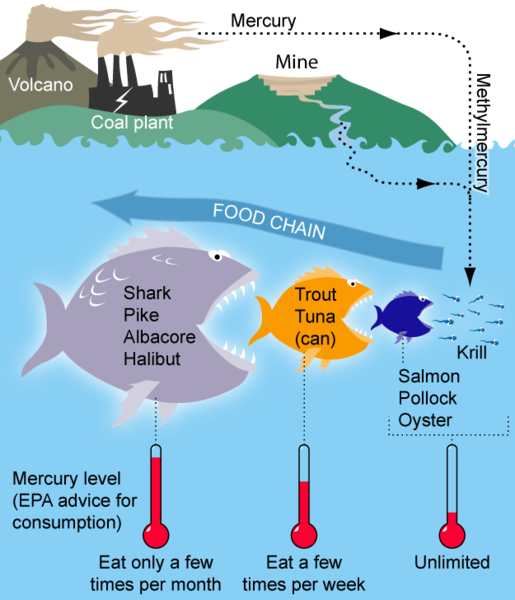
Basic diagram of how Mercury bioaccumulates and biomagnifies within a marine food chain, WikiMedia commons
Sharks are particularly susceptible to contamination uptake and bioaccumulation due to their long life spans, high position in a food web and large, lipid-rich livers (Mull et al., 2012). In the scientific paper, Heavy metals, trace elements, and organochlorine contaminants in muscle and liver tissue of juvenile white sharks, Charcharodon carcharias, from the Southern California Bight, by Mull et al., 2012, scientists were looking at toxic loads in white sharks and trying to determine if high levels of contaminants were caused by age/length or geographical trends. They found that high contamination levels in the sharks are directly linked with geographical areas that contained high levels of pollutants. Mull et al. also found that the average total mercury level in white sharks was six times higher than the established wildlife screening value of concern. These scientists also discovered that young of the year (YOY) white sharks from the Southern California Bight exhibited the highest liver levels of PCBs and DDT when compared to older white sharks or any other elasmobranch (sharks and rays) ever recorded! It is hard to believe that white sharks that are only one year old are carrying around enough toxins in their bodies that could cause sublethal effects including behavioral alterations, emaciation, cerebral lesions, and impaired gonad development (Wiener et al., 2003).

White shark toxin levels have been linked to geography and diet, making certain populations more susceptible to bioaccumulation. WikiMedia commons
In the paper Mercury bioaccumulation in the spotted dogfish (Scyliorhinus canicula) from the Atlantic Ocean by Coelho et al., 2010, scientists looked at the bioaccumulation pattern in different tissues in the spotted dogfish and the risks associated with its consumption because it is an ecologically important species. They found that the highest levels of mercury were in the muscle and that there was no significant difference in the concentrations between genders but was highest in the mature females. I found this to be very fascinating as I have heard several talks on toxic offloading and expected the opposite to occur. Toxic offloading is the process when a mother animal passes along toxins from its body to the developing offspring, thereby reducing its personal concentration levels. The key to this process is what type of reproduction the sharks possess. When a shark is ovoviviparous (eggs are produced inside the body but are then born via a live birth), there is limited mercury transfer because the eggs do not require extra nutrition during development. In viviparous species (live birth) there is pollutant transference along with the nutrients the mother sends to the embryos. Spotted dogfish are ovoviviparous and therefore the mother retains the majority of the toxins.
Sharks are not the only group that has to worry about toxic offloading. Marine mammals have had adverse effects to bioaccumulation and toxic offloading. In Haraguchi et al., 2009, Accumulation and mother-to-calf transfer of anthropogenic and natural organohalogens in killer whales (Orcinus orca) stranded on the Pacific coast of Japan, scientists tested the blubber of nine stranded killer whales and found that the concentrations of pollutants was higher in calves than lactating females. This indicates that large quantities of anthropogenic and natural persistent organohalogens (like DDT and PCBs) we being transferred from the mother to the calf via lactation. Now new born calves are starting with these high toxin loads and the effects of these elevated concentrations on the animal’s health and reproduction is unknown but may have been one of the causes of the strandings.

I know this all seems very “doom and gloom” but the more people are aware of these issues, the sooner we can make positive changes for our oceans and our health. One the easiest ways to avoid bioaccumulation of toxins in your seafood is to educate yourself about safe options. I love Monterey Bay Aquarium’s “Sustainable Seafood Watch Guide” which can be found on their website at http://www.montereybayaquarium.org/cr/cr_seafoodwatch/download.aspx. There is also an iPhone app, which is really convenient. Once you pick a region, you can view the best choices in sustainable seafood and which types of seafood are prone to mercury and other contaminants. You will see that some of the best choices have the little red star indicating possible contamination. These fish are to be limited in the quantity consumed by individuals per month. Sustainable seafood does not mean stop eating seafood, but rather a guideline towards making healthy choices for yourself and our oceans. Just like in the paper Mull et al., 2012, knowing where your seafood originates from can make all the difference when trying to determine seafood pollution levels. Different areas where seafood is harvested have varying types and levels of pollutants. Obviously the best option would be to stop the contaminants from entering the ocean in the first place but since a lot of these toxins originate as byproducts from consumer goods, it is easier to use the “3 R’s,” reduce, reuse and recycle. I could go on and on about ways to help the oceans but I hope this blog post has inspired you to do some research of your own and find at least one simple but effective way to make a difference. Every little bit counts.
REFERENCES
Coelho, J. P., Santos, H., Reis, a T., Falcão, J., Rodrigues, E. T., Pereira, M. E., Duarte, a C., et al. (2010). Mercury bioaccumulation in the spotted dogfish (Scyliorhinus canicula) from the Atlantic Ocean. Marine pollution bulletin, 60(8), 1372–5. doi:10.1016/j.marpolbul.2010.05.008
Haraguchi, K., Hisamichi, Y., Endo, T. (2009). Accumulation and mother-to-calf transfer of anthropogenic and natural organohalogens in killer whales (Orcinus orca) stranded on the Pacific coast of Japan. The Science of the total environment, 407(8), 2853–9. doi:10.1016/j.scitotenv.2009.01.003
Mull, C.G., Blasius, M.E., O’Sullivan, J.B., Lowe, C.G. 2012. Heavy metals, trace elements, and organochlorine contaminants in muscle and liver tissue of juvenile white sharks, Carcharodon carcharias, from the Southern California Bight. In Global perspectives on the biology and life history of the white shark (pp. 59-75). Domeier, M.L. Boca Raton, FL: CRC Press.
Wiener, J.G., Krabbenhoft, D.P., Heinz, G.H., Scheuhammer, A.M. 2003. Ecotoxicology of mercury. In Handbook of ecotoxicology, second edition (pp. 409-463). Hoffman, D.J., Rattner, B.A., Burton, G.A. Jr., Cairns, J., Jr. (eds.). Boca Raton, FL: CRC Press.