Olive Ridley Arribadas
by Daniela Escontrela, RJD Intern
Olive Ridley sea turtles, also known by their scientific name of Lepidochelys Olivacea, are as their name suggests an olive color. They have a heart shaped carapace (or top shell) and have either one or two claws on their flippers. These turtles tend to reach sexual maturity around 15 years of age, which is relatively young compared to other species of sea turtles; once they reach sexual maturity they nest about once or twice per season laying on average about 100 eggs per clutch. (NOAA 2013) Olive Ridleys are the most abundant sea turtles in the world, with 800,000 females nesting annually; however, they are listed as vulnerable under the International Union for Conservation of Nature. (Valverde et al 2012) In fact, the population of Olive Ridleys has seen a decline of about 60% since the 1960s. (NOAA 2013).
These turtle seem like any other species of turtle; they’re born, swim out to sea, come back and lay their nest, go back to sea and repeat this cycle over the course of its life. However, this genus of turtles (which includes the Olive Ridley and Kemp’s Ridley) demonstrate a particularly interesting behavior not observed in any other turtles; in fact this behavior can be considered a spectacle of nature. These turtles participate in something known as an “arribada”. An arribada is a mass nesting behavior were tens of thousands to hundreds of thousands of sea turtles come onto the beach to nest over the course of a couple of days. For most people, a nesting event is considered an arribada when more than 100 turtles are on the beach at the same time. (Valverde et al 2012) Although Olive Ridleys nest in over 40 countries, arribadas are only observed in a few countries: Mexico, Nicaragua, Costa Rica, Panama and India. No one really knows why these events happen or how the turtles know when it’s time to come ashore in mass. Some theories as to what triggers these events include offshore winds, lunar cycles and release of pheromones by females, but much research remains to be done. (NOAA2013)
Over the summer, I had the pleasure of traveling to Costa Rica, and as luck would have it, I had the opportunity to spend two weeks in Nancite beach, one of the beaches in Costa Rica were arribadas occur. The beach is located in Santa Rosa National Park and permits are required to go there. It is in an extremely remote location; in fact, to get there from the capital (San Jose) we had to take a four hour bus ride, then a two hour jeep ride and on top of all that a very laborious two hour hike. Nancite beach is small, only about one kilometer long, but regardless, during an arribada thousands of females come ashore to nest. Arribadas seem like a godsend for yet another species that is suffering from declines in population numbers; but arribadas aren’t as beneficial as they seem. When too many female turtles come to nest at once, nests that were already laid tend to be dug up by other females as they make their own nests. In addition, hatchling survival rate is very low and this may play a crucial role in the observed decline of nesting females at Nancite beach and the reduced frequency of arribadas. In fact, research revealed many nests showed no evidence of embryonic development. This may be due to high embryonic mortality at early stages or because a lot of the eggs are infertile, which could be explained by the low observations of mating in front of Nancite. (Valverde et al 1998) Some other challenges and reasons for decline will be discussed a little later.
Another arribada beach in Costa Rica is Ostional Beach which is also located on the northwest coast of Costa Rica. It was deemed a wildlife refuge 1983; in comparison to Nancite, this beach is a lot bigger, about seven kilometers. This important rookery was discovered in 1970 and it’s the second largest arribada beach in the world, second only to the one in La Escobilla, Mexico. What makes this beach different, is that it is the only beach in Costa Rica were harvesting of eggs is allowed. The small town of Ostional only has about 450 residents and since the legalization of egg harvesting in 1987, the people of Ostional have been doing just that. This legalization wasn’t without foundation; just like in Nancite Beach, a lot of egg clutches were being destroyed by other females during arribadas and hatchling success rates were very low, so maybe if some of the eggs were harvested, there would be a better chance of survival for the other clutches. In addition, legalization could help with the previously illegal take of eggs. So the community founded the Association for Integral Development at Ostional (the acronym in Spanish is ADIO). With the program, associates would harvest during the first 2.5 days of an arribada event and in return they would keep the beach clean and reduce the impact of feral predators. The eggs are sold all over the country and the proceeds go to both the associates and the community members. The problem with the program is that there seems to be no research to indicate the viability of the program; in fact in the past two decades, the amount of females that lay eggs at Ostional has decreased. (Valverde et al 1998)
Before we can jump to conclusions and blame the harvesting of eggs for the declines seen in both Ostional and Nancite, other factors have to be taken into account. Like mentioned before, arribadas aren’t as good as they seem, for one, females tend to destroy other nests as they build their own. The low hatchling success rates are another reason for concern. The low rates of success may be due to the high amounts of microorganisms that are related to the large number of clutches laid during arribadas. As Valverde explains “microorganism proliferation is thought to be a result from the increased amount of egg derived organic matter found in these beaches as a direct consequence of nest destruction by other nesting females”. Because of the high amounts of decomposing organic material from the broken eggs, oxygen levels also decrease which would create an anoxic environment for the eggs and hence prevent growth and development. It is possible that the egg harvest could be of crucial help as it would be removing eggs that otherwise would be destroyed and therefore decompose in the sand, deteriorating the conditions for the other surviving eggs clutches. (Valverde et al 1998)
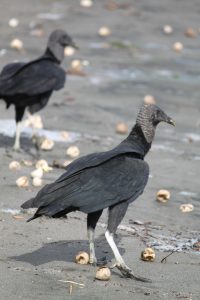
At Nancite beach, many eggs are washed out during high tides; egg clutches are also highly predated on. The removal of these eggs may help better the quality of the sand for the other eggs that are left (Photo taken by Dani Escontrela)
Olive Ridleys differ from other sea turtles in another way, they are highly nomadic. While most other turtles follow, to a certain extent, specific routes between their foraging grounds and nesting grounds, Olive Ridleys do not. Olive Ridleys have been tracked mostly in pelagic waters and have been found as far north as Mexico and as far south as Peru. There isn’t one exact place where this species goes for food, in fact it is believed that this turtles eat as they swim, they are opportunistic feeders. (Plotkin 2010) These migratory patterns can make them especially susceptible to overfishing in different countries where there may be no laws against the capture of sea turtles. In fact for a long time, the Olive Ridleys were fished at high rates by the Ecuadorian and Mexican fisheries; fortunately, fishing of sea turtles was banned in 1981 and 1990 respectively. Another danger faced by the Olive Ridley is the incidental mortality in shrimp nets, in which case turtles aren’t the target species of the fishery but are instead caught as by catch. In 1993 alone 60,000 turtles along the Pacific coast of Central American were killed by this method of fishing. Declines at both beaches can also be explained by beach exchange, were turtles change the beaches were they lay their nests. In fact, while I was in Nancite beach, we would often get Olive Ridleys that had been marked at Ostional beach. (Valverde et al 1998)
As grim as this all sounds, the future may not be so bleak for this species. As long as we put the time and effort we may be able to keep this species of turtle around. The fact that these turtles are nomadic doesn’t have to be a problem; fishing regulations need to be put in place in the countries the turtle frequents and also in international waters. However, there is actually a positive side to the turtles being nomadic too. Because the turtles show plasticity in their migration patterns, in times of environmental change, such as an El Nino event, the turtles would be able to move to different areas and find food. This means that Olive Ridleys might be more resilient when it comes to climate change. (Plotkin 2010) On another note, Valverde discovered in his research that Olive Ridley hatchlings showed the greatest success during the rainy season, in cooler temperatures and when clutch density is low. It is recommended then, that egg harvesting is restricted during these occasions to ensure the highest success for these hatchlings. (Valverde 2012)
The future also lies in continued research. We must keep evaluating and try to determine what is causing population declines at certain beaches and address these problems. In addition, more research needs to be done to figure out the viability of the egg harvesting program in Ostional; if it is deemed successful and viable, it should be continued and potentially be carried out in other arribada beaches with low hatchling success rates due to high density of clutches. All these efforts need to be taken not just by Costa Rica, but also by other countries where the Olive Ridley frequents seeing as the turtles are a shared resource and therefore should be protected by everyone.
REFERENCES
“Olive Ridley Turtle (Lepidochelys olivacea).” NOAA Fisheries Office of Protected Resources. N.p., 1 Mar. 2013. Web. 2 Oct. 2013. <http://www.nmfs.noaa.gov/pr/species/turtles/oliveridley.htm>.
Plotkin, Pamela T. “Nomadic behaviour of the highly migratory olive ridley sea turtle Lepidochelys olivacea in the eastern tropical Pacific Ocean.” Endangered Species Research 13.1 (2012): 33-40. Print.
Valverde, R. A., et al. “Olive Ridley Mass Nesting Ecology and Egg Harvest at Ostional Beach, Costa Rica.” Chelonian Conservation and Biology 11.1 (2012): 1-11. Print.
Valverde, Roldan A., Stephen E. Cornelius, and Claudette L. Mo. “Decline of the Olive Ridley Sea Turtle (Lepidochelys olivacea) Nesting Assemblage at Nancite Beach, Santa Rosa National Park, Costa Rica.” Chelonian Conservation and Biology 3.1 (1998): 58-63. Print.