A Scientific History of Oysters in Chesapeake Bay
By Nicole Suren, SRC intern
Oysters are not only a preferred dish of much of the human population, but they are also very important parts of the ecosystems they inhabit. As ecosystem engineers, or organisms that significantly modify their habitat, they do not just participate in the habitat they settle in but improve it by filtering large volumes of water and forming reefs that other organisms can use as shelter. Unfortunately, the estuary systems that they prefer have been in steep decline for some time due to negative effects of human activity, and scientists are currently attempting to quantify how much these ecosystems have declined by examining the average sizes of oyster populations over time. Here, Rick et al. (2016) focused on Chesapeake Bay in the northeastern United States.
Change in oyster size over time
There is an important relationship between the size of the oysters and how healthy their populations are. Generally speaking, larger average oyster size correlates with a healthier and more abundant oyster population. With this in mind, scientists examined fossilized oysters ranging from 1,500-3,000 years ago, oysters from Native American archaeological sites, and modern oysters to see how the introduction of humans and later new fishing technologies would affect the oyster populations. They found that the prehistoric oysters were the largest, and that the introduction of harvesting by Native American populations did not affect the average size of the oysters. However, the introduction of new fishing technology resulted in an increase in oyster size, but this then decreased to a much smaller average size than the prehistoric samples today.
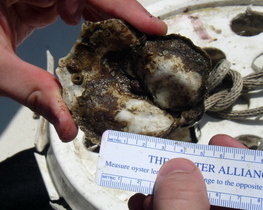
Measurements of Chesapeake Bay oysters taken by NOAA
What do these changes mean?
Several things about these results are important. First, the increase in oyster size upon the advent of new fishing technology can be attributed to people having access to parts of the oyster population that they had not had access to previously. The Native Americans were believed to harvest oysters by hand, so this would have limited them to slightly smaller oysters in shallow waters. In contrast, new fishing techniques such as trawling would have harvested the larger oysters in deeper waters, so the size increase is likely due to a difference in sample areas between the two time periods. Second, since there was no size difference between the oyster populations before and after Native American settlement in Chesapeake Bay, it can be concluded that the Native American oyster fishery was very sustainable. This is hypothesized to be due to their aforementioned harvesting methods, low population density, broad-spectrum diets (that are not completely dependent on oysters), and lack of a significant oyster trade.
Implications for current management
While it would be impossible to fully emulate the sustainability of the Native Americans of that time, we can use the same principles to modify our own activities to make the modern oyster fishery more sustainable. For example, fishing limits on larger oysters in deeper waters, decreased harvests of all oysters, and restoration techniques can be implemented, all of which mirror Native American sustainability strategies in a modern world. The implications of this study show that science is deeply rooted in cultural phenomena, and can incorporate the best of culture and sustainability to better the planet.
Reference
Rick TC, Reeder-Myers LA, Hofman CA et al. (2016) Millennial-scale sustainability of the Chesapeake Bay Native American oyster fishery. Proceedings of the National Academy of Sciences, 113, 6568–6573.