Historical vulnerability of manatees to boat strikes in Florida
By Haley Kilgour, SRC MPS student
Manatees are an iconic species of Florida and for many years they have been one of the main focuses of conservation. Placed on the endangered species list in 1967, manatees have managed to make a comeback from their population containing only a few hundred individuals to over 6,000 (2016 count) (Gast, 2017). Now, as of March 31, 2017 Manatees have been “downlisted” to threatened (Gast, 2017). But that does not mean their troubles are over.
There are three major criteria for the downlisting of a species: 1) population must be growing or stable, 2) mortality factors must be decreasing or controlled at acceptable levels, and 3) habitats must be secure (Marsh and Lebefvre, 1994). As it stands, manatee populations have increased enough to meet the first criterion, but the second statue is not being met and the third criteria can be a hit or miss, depending on the location you are referring to. While still protected by the Marine Mammal Protection Act (1972) and the Endangered Species Act (1973) (Marsh and Lebefvre, 1994), hundreds of manatees are still killed by anthropogenic means. Roughly 30% of annual mortalities are from vessel collisions (Aipanjiguly, 2003 and Nowacek, 2004); of 520 deaths in 2016, 104 were from boats (Gast). Figure 1 demonstrates how many manatees are hit by boats. It is without a doubt that boat collisions are the number one reason for manatee deaths (Runge et al., 2007; Runge et al., 2016; Marsh and Lebefvre, 1994; Aipanjiguly, 2003; Baudin et al., 2012; Nowacek et al., 2004). While all manatee-boat collisions do not result in death, 97% of manatees bear scar patterns, which can be used to identify them (Nowacek et al., 2004). It must also be noted that mortality from collision can occur year round and involve all age classes (Nowacek et al., 2004).
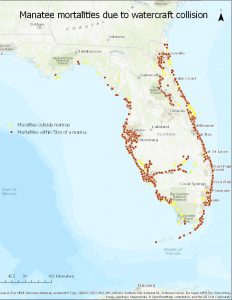
Figure 1: Manatee deaths caused by boat collisions, focusing mostly on deaths within 5 kilometers of marinas. Deaths shown are from 1976 –present.
Manatees are classified as a marine species but they require access to deep water and freshwater, and thus can be found in inland rivers, coastal estuaries, seagrass beds, and marinas (Marmontel et al., 1997). Manatees feed exclusively on seagrass and these beds are found almost entirely in publically owned waters (Marmontel et al., 1997). Bauduin et al. (2013) found that manatees do no avoid developed areas, so it is humans that need to change their practices if this species is to persist.
Nowacek et al. (2004) found that the best way to reduce manatee morality from anthropogenic causes is to reduce boat speeds in areas of high density. Something to note is that manatee protection zones have been implemented since 1979 (Edwards et al., 2016), but not much has changed as far as mortality from boat collisions. Possible reasons for this is that regulations are either not being enforced or that further restrictions are necessary (Nowacek et al., 2004). One excellent example of blatant disregard of regulation is seen in the Bear Cut protection zone where even police boats ignore the no wake zone. In situations like this more stringent law enforcement and education is required to remedy the problem.
It is important to decrease boat speeds in areas of high manatee density because the manatees need time to respond. Nowacek et al. (2004) found that manatees do not respond to boats until the vessel is on average about 25-50 meters away. Another problem is that in areas of high boat traffic, manatees have problems distinguishing multiple inputs. Even with conservation efforts well underway, the number of carcasses recovered each year is close to 200 (Marsh and Lebefvre, 1994). It is necessary to decrease the number of deaths from boat strikes because Runge et al. (2012) found manatee populations are still capable of dropping below a critical threshold within 50-100 years if current mortality patterns persist.
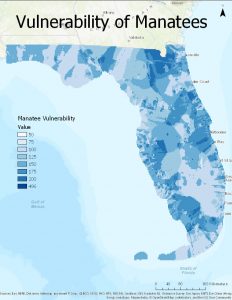
Figure 2: A map of manatee vulnerability based upon deaths near marinas.
With only four extant species of Sirenia, it is important to persevere these charismatic creatures. While it may be an overreach to want to prevent all manatee deaths from boat collisions, it is plausible to decrease the number. Creating more speed-limit zones will give manatees adequate time to avoid boats, which is one or the largest reasons they are hit. And for those that choose to ignore regulations, they need to face larger consequences from a law agency that enforces what they are supposed to. Education can also be key in helping people understand the reasons behind restrictions and the necessity to abide by them, as well as to increase awareness of manatees while operating watercraft.
References
Aipanjiguly, Sampreethi, et al. “Conserving Manatees: Knowledge, Attitudes and Intentions of Boaters in Tampa Bay, Florida.” Conservation Biology, vol. 17, no. 4, 2003, pp. 1098-1105
Baudin, Sarah, et al. “An index of risk of co-Occurance between marine mammals and watercraft: Example of the Florida manatee.” Biological Conservation, vol. 159, 2013, pp. 127-136.
Edwards, H. H, et al. “Influence of manatees’ diving on their risk of collision with watercraft”. PLoS One, 11. 2016
Gast, Phil. “It’s Official: Manatee no longer endangered.” CNN, Cable News Network, 31 Mar. 2017.
Marsh, H., and Lebefvre, L. W. “Sirenian status and conservation efforts.” Aquatic Mammals, vol. 20, no. 30, 1994, pp. 155-170.
Marmontel, Miriam, et al. “Population Viability Analysis of the Florida Manatee (Trichechus manatus latirostris), 1976-1991. Conservation Biology, vol. 11, no. 2, 1997, pp. 467-481.
Nowacek, Stephanie M, et al. “Florida manatees, Trichechus manatus latirostris, respond to approaching vessels.” Biological Conservation, vol. 119, no. 4, 2004, pp. 517-523.
Runge, Michael C., et al. “Status and threats analysis for the Florida manatee (Trichechus manatus latirostris), 2012.” Open-File Report, 23 Mar. 2007.
Runge, M.C., et al. “Status and threats analysis for the Florida manatee (Trichechus manatus latirostris)”, 2016.